Diagnostics to Go: Researchers Eye Wearable Heart-Scan Patch
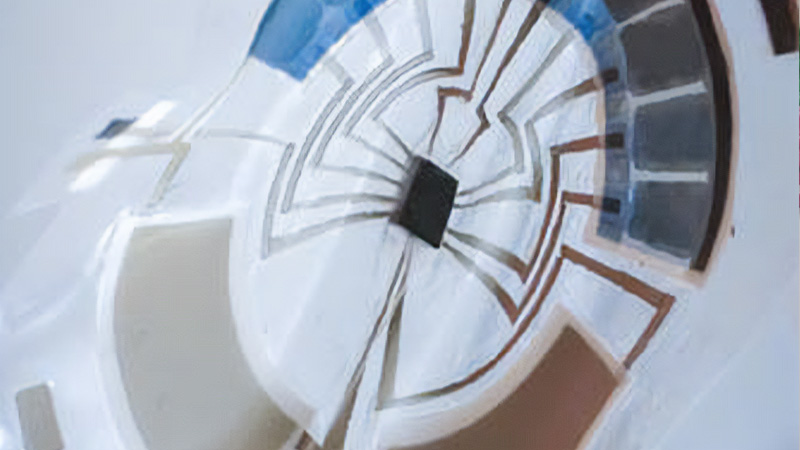
UC San Diego researchers use SEBS copolymer to develop a wearable ultrasound patch
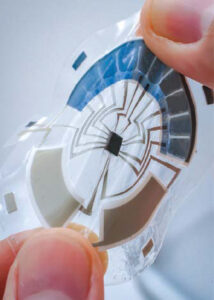
This thin, flexible and stretchy sweat sensor shows the level of glucose, lactate, sodium or pH of sweat at the press of a finger. Photos courtesy of University of California, San Diego
Researchers at the University of California, San Diego (UCSD), are using a styrene-based triblock copolymer to help enable the development of a wearable ultrasound patch that could offer real-time heart scans on the go. The material, a styrene-ethylene-butylene-styrene (SEBS) thermoplastic elastomer, helps to make the patch softer, more compliant and inherently stretchable.
This solves one of the major issues with existing ultrasound patches that rely on metal-based contacts, says Lu Yin, a chemical engineer and postdoctoral researcher in Prof. Joseph Wang’s nanoengineering lab at UCSD. Previous iterations of ultrasound arrays tended to use laser-cut thin metal serpentine interconnects that both increased the design’s complexity, suffered from irreversible deformations and were less effective at maintaining good contact with the skin to ensure stable connectivity.
Yin is the polymer-based composite materials expert on the team and he introduced the concept of using a processable, SEBS-based copolymer some two years ago to the UCSD lab, which focuses largely on developing printed electronics for various applications. The polymer helps to make the resulting devices stretchable he noted in a Jan. 27 telephone interview.
Ultrasound imaging (sonography) uses high-frequency sound waves to view inside the body. Because ultrasound images are captured in real time, they can also show movement of the body’s internal organs as well as blood flowing through blood vessels. But ultrasound devices traditionally have been bulky, meaning they are typically applied in a clinical setting.
This latest non-invasive device, which measures roughly one inch square, features piezoelectric transducer arrays, liquid metal composite electrodes and a triblock SEBS copolymer encapsulation. While it is a long way from commercialization, the researchers note that the technology potentially has multiple applications, to include scanning the spine, liver and veins, monitoring blood pressure and blood flow (e.g., in stroke patients) and being able to help athletes optimize their performance.
Overcoming Challenges
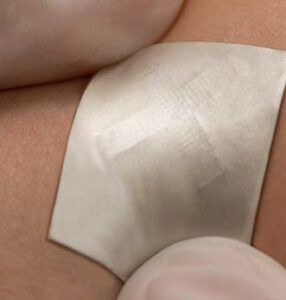
The SEBS-based ultrasound patch conforms and adheres firmly to the skin and measures barely 1 inch square.
For this project—headed by Hongjie Hu, a postdoctoral researcher in Prof. Sheng Xu’s research group at UCSD—the team says it exploited a new technology for patterning. “We first doctor blade-coated a thin layer of liquid metal on a substrate. A key consideration before printing was how to get the liquid metal to wet the substrate.” To address this issue, they dispersed big liquid metal particles into small microparticles using a tip sonicator. When microparticles contacted air, their outermost layer generated an oxide coating, which lowered the surface tension and prevented those microparticles from aggregating.
“In addition,” the team says, “we used 1.5 wt.% SEBS as a polymer matrix to disperse the liquid metal particles because SEBS could wet well on the liquid metal surface. We also used SEBS as the substrate. Therefore, the SEBS in the matrix and the substrate could merge and cure together after printing, allowing the liquid metal layer to adhere to the substrate efficiently and uniformly. Then we used laser ablation to selectively remove the liquid metal from the substrate to form patterned electrodes.”
They next bonded a SEBS film with a transducer element and conducted a lap shear test. The bonding strength between the pure SEBS film and the transducer element and between the electrode and the transducer element were both stronger than many commercial adhesives.
The SEBS material begins as layers that are 200 microns thick, but then, using a solvent process, “we dissolve SEBS to make a thick resin, and then cast it onto a supportive backing” that is later removed. Once the material dries, it becomes about one-fifth of its original thickness, because the solvent has all dissipated, Yin said.
“On top of that we apply a layer of liquid metal/SEBS composite, which is the ink we developed. And then a laser is used to cut away part of the liquid metal that is not wanted. This exposes the traces for the [electrical] connection.” By repeatedly applying the SEBS layer and the liquid metal composite layer followed by laser ablation, the multilayered, stretchable circuit can be made, he explained.
“We’ve developed a process to mount the rigid components, like an integrated circuit chip or a rigid ultrasound transducer, which is a ceramic material,” Yin said. “So, we use a very low-temperature-based process—a ‘solvent soldering’ process, we call it. It is to make the substrate or ink partially redissolve or swell and while it is re-crosslinking back into its polymer form, it also grabs the metal part with it. So essentially with a drop of solvent you can achieve what people would need to do with high-temperature soldering, but now it’s compatible with these kinds of thermoplastic materials.”
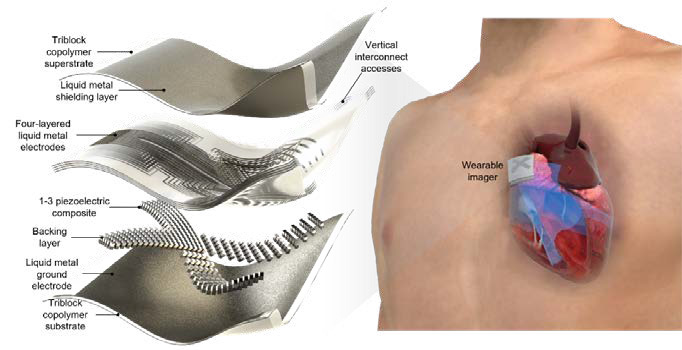
Sandwiched between flexible layers of triblock copolymers, the patch is far from commercialization, but holds great promise for medical monitoring.
Stretchy Sweat Sensor
The UCSD team also revealed last fall that it has developed a thin, flexible and stretchy sweat sensor that can show the level of glucose, lactate, sodium or pH of sweat, all at the press of a finger. Claiming it reflects the new state-of-the-art of wearable sensors, the researchers call it “the first standalone, wearable device that allows the sensor to operate independently, without any wired or wireless connection to external devices, to directly visualize the result of the measurement.” The researchers disclosed their findings about this fully integrated soft skin patch in a paper published Oct. 6, 2022, in Nature Electronics.
They say the unique design of this small, disk-shaped patch includes all the essential components required for wearable sensors—two integrated batteries, a microcontroller, sensors, the circuit and a stretchable display. This covers all functionalities essential for operating a wearable sensor, from powering up to showing a user the results.
“We are trying to address the issue of practicality in wearable technologies,” said Yin, one of the paper’s authors. “We have seen so many inventions of wearable sensors with great novelty but with limited practicality.”
Yin said the work “requires every trade of engineering to come together. You need experts in sensors, displays, batteries, circuit designs and firmware engineering to get every module within this small patch to work. The integration of them also requires the knowledge in electrochemistry, electronic engineering and material science to ensure that all parts remain stretchable, reliable and compatible to work seamlessly together as a system.”
The fabrication of the device involves the formulation of nine types of different stretchable inks, which were used to print the batteries, circuits, display panel and sensors. The device is printed layer-by-layer onto stretchable polymer sheets and then assembled with hydrogels and microcontroller chips into the complete device. The team optimized each ink to ensure its compatibility with other layers while balancing its electrical, chemical and mechanical performance.
“Adding the flexible display and stretchable battery has a tremendous impact toward practical epidermal microscale sensing platforms,” said Prof. Wang, a senior author of that paper and co-director of the UCSD Center for Wearable Sensors.
To visualize the data from the sensors on that sweat-analyzing patch, the team designed a special type of nonlight-emitting display called electrochromic display. The electrochromic display technology uses materials that change color upon applying an electrical pulse, which has very low power consumption.
“Typical electrochromic displays require transparent glass panels with a conductive but brittle coating, which does not work for our device,” Yin said. Instead, the researchers used a special polymer called PEDOT:PSS, which is both conductive and has electrochromic properties. The polymer changes from light sky blue to dark navy blue when applying a negative voltage and turns back when applying a positive voltage. “By tuning the ink formulation with PEDOT:PSS, we can make it both printable and stretchable,” he explained.
PEDOT:PSS, a poly(3,4-ethylenedioxythiophene) polystyrene sulfonate, is a polymer mixture of two ionomers. One component in this mixture is made up of polystyrene sulfonate, which is a sulfonated polystyrene. Part of the sulfonyl groups are deprotonated and carry a negative charge. The other component, poly(3,4-ethylenedioxythiophene) (PEDOT), is a conjugated polymer and carries positive charges and is based on polythiophene. Together the charged macromolecules form a macromolecular salt.
Keys to Market
A separate spin-off company at UCSD is working to commercialize the lab’s technologies, Yin said. Such work is beyond the scope of university lab researchers. Much remains to be done in terms of investment and further collaboration before their technology can be transformed into a very sophisticated and commercially ready medical device, Yin acknowledged.
As for the ultrasound patch, the next step is to develop a way to integrate the back-end electronics for signal processing and power supply. These ultrasound machines generate very high band-width data, so a strong processor and data transmission scheme will be needed to handle the data demands in real time. Related work is under development and may be available in publication soon.
“The sensor is just the part that generates the raw data, but it also needs to be connected to an entire, integrated system before it can be a usable commercial tool,” Yin explained. It needs a wire to come out and connect to an instrument to sample the data, and then processing software is needed to yield the desired images.
“I’m very bullish on SEBS,” he added. “It is being used more and more in biomedical applications. You see it used as a coating on catheters, or to accommodate shape-changing sensors. SEBS has been used to wrap around a nerve, so as the monitored subject grows, the sensor can grow with the tissue.”
Yin said his lab is also working closely with Prof. Xu’s lab at UCSD to integrate chemical sensors with physiological sensors so they can do multiplex sensing of many things. As for the imaging part, that can be used to image structures and different tissues, blood flow, the contraction of muscles, etc.
“The application [of this technology] is endless,” Yin said. “It’s an imaging tool. How you use it will be up to your imagination. There is a lot of potential.”